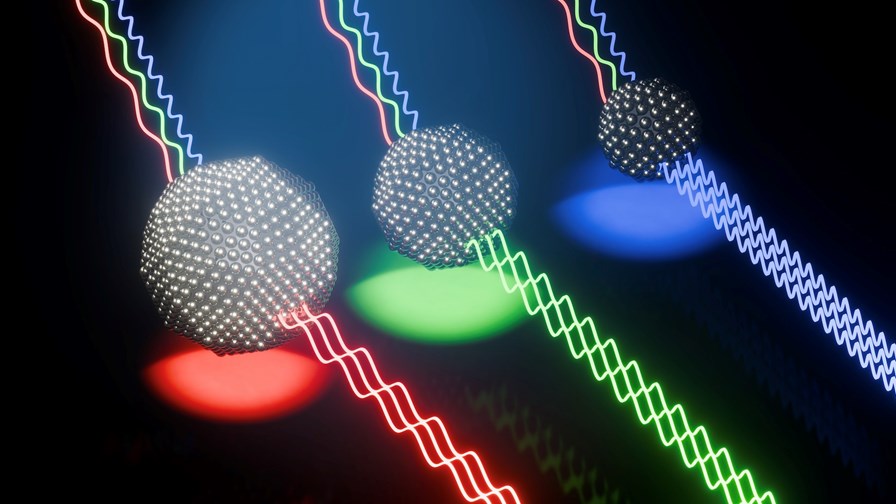
- Quantum dots tuned to C-Band telecoms frequencies will be compatible with existing fibre-optic telecoms networks
- This offers enormous potential for global quantum telecoms networks and the quantum internet of the future
- Tiny semiconductor particles can, according to their size, emit any colour of light
- Indistinguishable photons to enable scalable schemes of quantum comms
At some point, maybe in 10 years or so but more likely within a generation, quantum telecoms and the quantum internet should be the global norm for the generation, distribution, storage and processing of data via quantum bits (qubits) linked across different quantum nodes via quantum states of light.
To work across fibre-optic networks and within the bounds of accepted, standardised methodologies and compatibilities (not to mention the immutable laws of physics), long-distance quantum telecoms networks will need quantum repeaters operating in telecoms frequencies. Semiconductor quantum dots (QDs) show great promise in this regard.
Discovered back in 1980, quantum dots are incredibly small semiconductor particles ranging in diameters of between 2 and 10 nanometres. They have a unique optical property in that they can absorb light energy, achieve an ‘excited’ state, and then release photons as they return to their original ‘ground’ state. According to their size, quantum dots fluoresce to emit light of different colours when light energy is applied to them.
Generally, the smaller the QD, the shorter the emission wavelength. Also, the smaller the QD, the bigger the “minimum band gap”, which is the amount of energy needed to excite an electron into a state of enhanced energy. Thus, smaller QDs require more energy for excitation and emit lower wavelengths of light, because the wavelength is inversely proportional to the excitation energy applied.
Concurrently, more energy is released when the crystal returns to its original ground state. This results in a colour shift from red to blue in the emitted light. It means nanomaterials can potentially emit any colour of light from the same material simply by changing the dot size, and the semiconducting materials can be ‘tuned’ to each colour during the manufacturing process or even when in the operational system itself.
Earlier this year the US National Library of Medicine published a paper, On-Demand Generation of Indistinguishable Photons in the Telecom C-Band Using Quantum Dot Devices, detailing the progress of research into the solving of problems associated with long-distance quantum information transfer via optical fibres. It reports that it has been determined that “wavelengths around 1550 nm, [that is, in the telecom C-band which covers 1530-1565 nm] are required to benefit from the lowest losses in optical fibres.”
It adds, “To shift the emission of QDs to C-Band wavelengths, quantum frequency conversion of QD-photons emitted at shorter wavelengths can be used which, however, introduces additional conversion losses, ultimately limiting the source efficiency. For this reason, QDs directly emitting photons at telecom wavelengths are desirable, requiring carefully tailored growth schemes.”
Distinguishing the indistinguishable
Meanwhile, another academic paper, Indistinguishable single photons with flexible electronic triggering, from the Optica Publishing Group, tells us that, for a considerable number of years now, “photons generated on-demand using QDs have demonstrated unprecedented quantum optical properties in terms of high purity, brightness, and indistinguishability and have been repeatedly employed in implementations of quantum communication.”
The notion of “indistinguishability” is important because single photons are the prime candidates for the realisation of scalable schemes of quantum communication and of linear optical quantum computing. Why? “Because, in each instance, the performance of such schemes is critically dependent on the indistinguishability of the single photons in key applications such as quantum repeaters.” A high degree of “indistinguishability” requires almost complete wave-packet overlap, or perfect photon matching of energy, space, time and polarisation.
The paper continues, “Of the various types of single-photon sources and semiconductor, QD are regarded as very promising for generating indistinguishable single photons because they offer a robust platform in which a single quantum system can be embedded in semiconductor devices and designed into bright single- and entangled-photon sources. The ideal single-photon source for quantum information processing (QIP) applications is one that generates a pure single-photon Fock state on demand ie. in response to an independent trigger signal from a user.” In quantum mechanics, a Fock state (or number state) is a quantum state that is an element of a Fock space with a well-defined number of particles. Fock states are named after the Soviet-era physicist, Vladimir Fock.
Finally, another report, Purcell-enhanced single photons at telecom wavelengths from a quantum dot in a photonic crystal cavity published just a week ago in Nature magazine, has it that, “Quantum dots are promising candidates for telecom single photon sources due to their tunable emission across the different low-loss telecommunications bands, making them compatible with existing fibre networks. Notably, semiconductor QDs may be engineered to emit over a large spectral emission window, ranging from the visible to the technologically relevant telecommunications C-band.”
It adds, “The potential for operation in the low-loss C-band spectral window for standard silica fibre provides strong motivation for their continued development. Such a source would be compatible with existing fibre networks, with the potential for long distance fibre-based quantum communication, as well as low-loss connectivity between individual nodes of the future quantum network. High repetition-rate single photon sources at telecommunications wavelengths can enable high bit-rate quantum communications over fibre networks.”
By the way, back in the 1940’s Edward Mills Purcell, who won the 1952 Nobel Prize for Physics, found that the spontaneous emission rates of atoms are enhanced when incorporated into a resonant cavity. An optical cavity (also known as a resonating cavity or optical resonator) is an arrangement of mirrors and/or other optical elements that forms a cavity resonator for light waves. They are a major component in lasers.
– Martyn Warwick, Editor in Chief, TelecomTV
Email Newsletters
Sign up to receive TelecomTV's top news and videos, plus exclusive subscriber-only content direct to your inbox.